Source-ACS
The idea of polymorphs is an intriguing mystery in the field of materials science, providing deep insights into the variety and adaptability of crystalline structures. Allotropes, another name for polymorphs, are several crystalline forms of the same substance, each with unique chemical and physical characteristics. They are found in many different materials, from graphite to diamonds and beyond, and they influence both scientific and industrial endeavors. Within this piece, we set out to explore the intricacies of polymorphs, exploring their importance, traits and uses in a variety of fields.
Understanding Polymorphs
Polymorphs, derived from the Greek words “poly” (meaning many) and “morph” (meaning form), represent a phenomenon wherein a single chemical compound can exist in multiple crystal structures under different conditions of temperature, pressure, or other environmental factors. This inherent variability gives rise to diverse polymorphic forms, each exhibiting unique arrangements of atoms or molecules within the crystal lattice.
This fascinating phenomenon of polymorphism highlights the dynamic nature of materials and underscores the intricate relationship between their structure and properties. The ability of a substance to adopt multiple crystal structures reflects the delicate balance between thermodynamic stability and kinetic accessibility, offering a glimpse into the complexity of molecular interactions at the atomic level.
Furthermore, the existence of polymorphs provides valuable insights into phase transitions, solid-state transformations, and the underlying mechanisms governing material behavior. By unraveling the mysteries of polymorphism, scientists aim to unlock new avenues for materials design, functional optimization, and technological innovation, paving the way for groundbreaking discoveries and advancements across various disciplines.
The Intricacies of Polymorphism
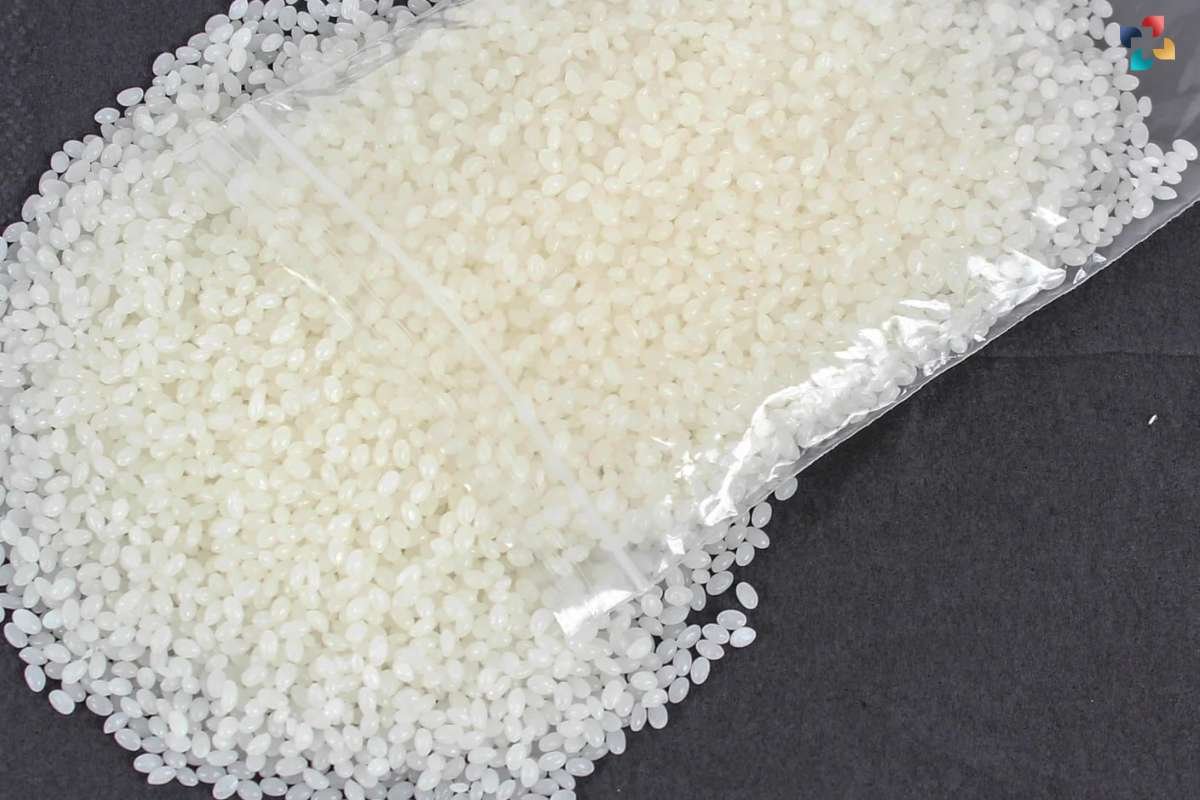
The polymorphic behavior of a substance arises from the intricate interplay between thermodynamics, kinetics, and molecular interactions. At the heart of polymorphism lies the concept of energy minimization, wherein different crystal structures compete to achieve the most stable configuration under specific conditions. Factors such as molecular symmetry, packing efficiency, and intermolecular forces dictate the formation of polymorphs, leading to a rich tapestry of crystalline diversity.
Also Read: Deciphering the Role of Single Nucleotide Polymorphism (SNP) in Genomic Diversity
Exploring Polymorphic Transformations
Polymorphic transformations, wherein a substance transitions from one crystal form to another, are governed by complex thermodynamic and kinetic processes. These transformations can occur spontaneously due to changes in temperature or pressure, or they may be induced through external stimuli such as mechanical stress or solvent interactions. Understanding the mechanisms underlying polymorphic transitions is essential for predicting material behavior and designing novel crystalline forms with tailored properties.
Polymorphic transformations represent intricate phenomena influenced by a myriad of factors, including molecular structure, intermolecular forces, and environmental conditions. The dynamic nature of these transformations often results in the emergence of new crystal structures with unique physical and chemical properties, offering opportunities for materials optimization and innovation. Through advanced analytical techniques and computational modeling, scientists strive to unravel the complexities of polymorphic transitions, shedding light on the underlying thermodynamic pathways and kinetic barriers involved. By gaining deeper insights into these mechanisms, researchers can manipulate polymorphic transformations to engineer materials with tailored functionalities, driving advancements in fields ranging from pharmaceuticals to electronics and beyond.
Applications of Polymorphs
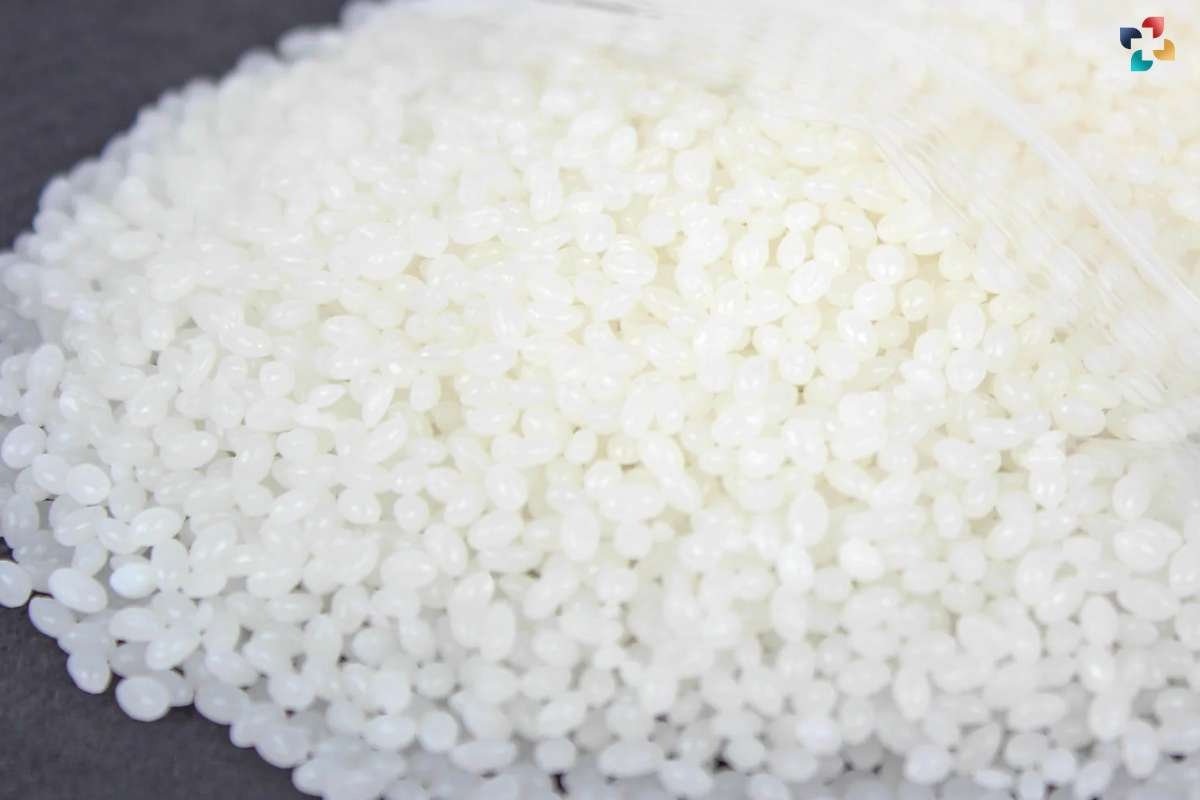
The significance of polymorphs extends far beyond academic curiosity, permeating various industries and technological advancements. In the pharmaceutical sector, for instance, polymorphs play a pivotal role in drug development and formulation. Different polymorphic forms of a drug molecule can exhibit distinct solubility, bioavailability, and stability profiles, influencing its therapeutic efficacy and commercial viability. By selecting the most suitable polymorph, pharmaceutical scientists can optimize drug performance and ensure consistent product quality.
In the realm of materials engineering, polymorphs offer opportunities for tailoring material properties to meet specific requirements. For example, in the field of solid-state electronics, the choice of polymorphic form can significantly impact the electrical conductivity, mechanical strength, and optical properties of semiconducting materials. By harnessing the polymorphic diversity of materials like silicon and carbon, researchers can innovate novel technologies ranging from high-performance transistors to next-generation photovoltaic devices.
Moreover, polymorphs hold promise in the realm of energy storage and conversion, where materials with optimized crystal structures can enhance the efficiency and durability of batteries, fuel cells, and catalysts. By exploring the polymorphic landscape of materials such as lithium-ion battery electrodes or hydrogen evolution catalysts, scientists aim to unlock new avenues for sustainable energy generation and storage.
Challenges and Future Directions
Despite their immense potential, the study and exploitation of polymorphs are not without challenges. The characterization of polymorphic forms, for instance, often requires sophisticated analytical techniques such as X-ray diffraction, solid-state NMR spectroscopy, and computational modeling. Additionally, controlling polymorphic transformations and understanding their impact on material performance demand interdisciplinary approaches integrating chemistry, physics, and materials science.
Looking ahead, the field of polymorphs holds immense promise for innovation and discovery. Advances in computational methods, high-throughput screening techniques, and crystal engineering approaches are poised to revolutionize our understanding of polymorphic phenomena and accelerate the development of novel materials with tailored properties. By harnessing the inherent variability of polymorphs, researchers can unlock new frontiers in drug design, materials synthesis, and technological innovation, paving the way for a brighter and more resilient future.
Conclusion
To sum up, polymorphs are evidence of the extraordinary variety and flexibility of crystalline materials. They are fundamental to materials science and engineering because of their complex atom-to-atom interactions within crystal lattices and their far-reaching effects on several sectors. Scientists and inventors have the opportunity to usher in a new era of discovery and advancement, profoundly and transformatively influencing the world of tomorrow by deciphering the mysteries of polymorphism and using its potential.
FAQs:
1. What are polymorphs in materials science?
Polymorphs are different crystalline forms of the same substance, exhibiting distinct arrangements of atoms or molecules within the crystal lattice. These variations in structure can result in differences in physical and chemical properties.
2. How do polymorphs differ from allotropes?
While both polymorphs and allotropes refer to different forms of the same substance, polymorphs specifically describe variations in crystal structure, whereas allotropes encompass variations in molecular structure. For example, carbon exists in various allotropes like diamond, graphite, and graphene, while different crystal forms of the same substance, such as quartz and cristobalite, are considered polymorphs.
3. What factors influence the formation of polymorphs?
The formation of polymorphs is influenced by factors such as temperature, pressure, solvent interactions, and molecular packing efficiency. Changes in these parameters can lead to alterations in the energetics of crystal formation, resulting in the emergence of different polymorphic forms.
4. Why are polymorphs important in pharmaceuticals?
Polymorphs play a critical role in pharmaceuticals as they can significantly affect drug solubility, bioavailability, and stability. The selection of the appropriate polymorphic form is crucial for ensuring consistent drug performance, formulation efficacy, and regulatory compliance.
5. How can polymorphs be characterized and identified?
Polymorphs can be characterized and identified using various analytical techniques, including X-ray diffraction, solid-state NMR spectroscopy, differential scanning calorimetry, and microscopy methods. These techniques provide valuable information about the crystalline structure, thermal behavior, and physical properties of polymorphic forms, aiding in their characterization and classification.