One essential mechanism that keeps life going is cellular respiration, which gives cells the energy they need to perform a variety of tasks. The complex metabolic pathway that produces adenosine triphosphate (ATP), the cellular energy currency, entails breaking down organic compounds. Deciphering the intricate network of energy production within cells requires an understanding of the stages and mechanisms of cellular respiration.
Glycolysis, the citric acid cycle (Krebs cycle), and oxidative phosphorylation (electron transport chain and chemiosmosis) are the three primary phases of cellular respiration. Every phase adds to the total conversion of nutrients into ATP, demonstrating the remarkably high production efficiency of cellular energy.
Exploring the Intricacies and Implications of Cellular Respiration:
1. Glycolysis: Breaking Down Glucose for Energy
Glycolysis initiates cellular respiration by breaking down a molecule of glucose into two molecules of pyruvate. This anaerobic process occurs in the cytoplasm and involves a series of enzymatic reactions. Despite its simplicity compared to subsequent stages, glycolysis is a crucial step, providing a starting point for both aerobic and anaerobic respiration.
During glycolysis, glucose undergoes a series of transformations, producing ATP and nicotinamide adenine dinucleotide (NADH). While glycolysis yields a modest amount of ATP directly, its primary significance lies in preparing the pyruvate molecules for further processing in the citric acid cycle.
2. The Citric Acid Cycle: Unveiling Energy-Rich Molecules
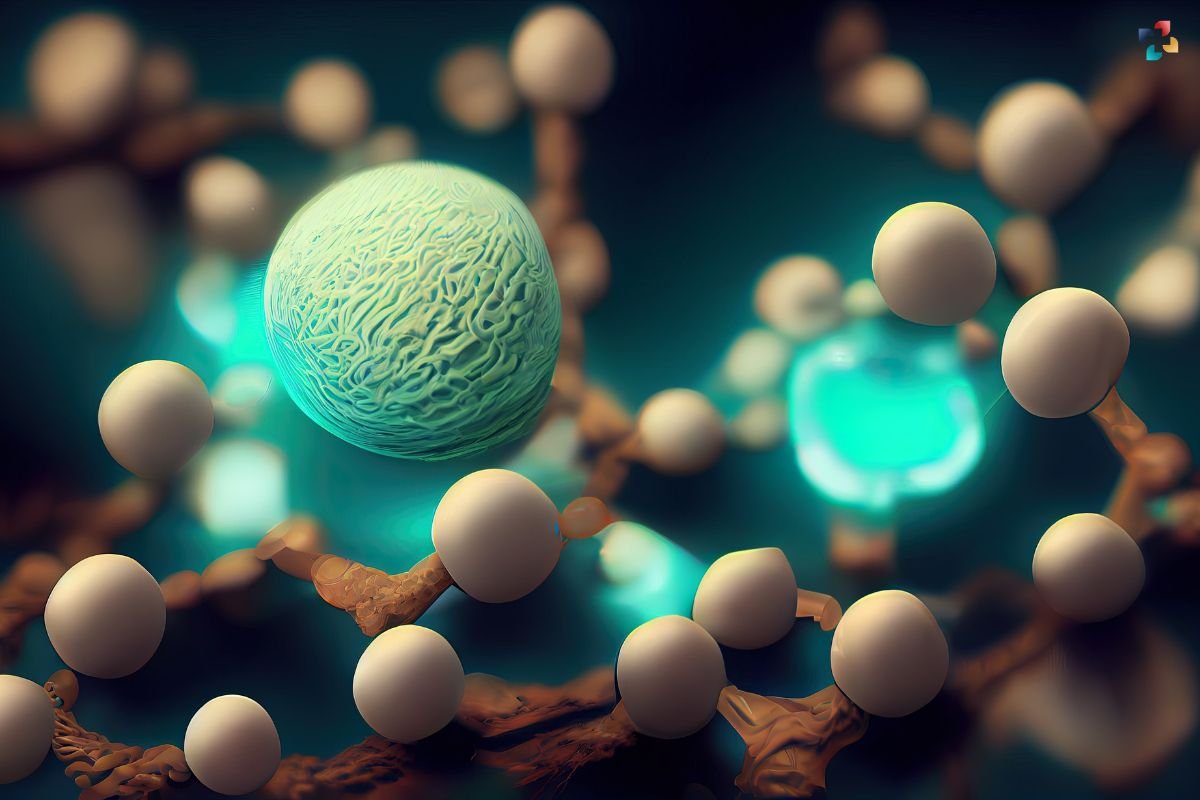
Following glycolysis, pyruvate enters the mitochondria, where the citric acid cycle extracts high-energy electrons. This cycle, also known as the Krebs cycle, involves a series of enzyme-driven reactions that release carbon dioxide while generating NADH and flavin adenine dinucleotide (FADH2). Additionally, the cycle produces ATP through substrate-level phosphorylation.
The citric acid cycle is a pivotal link between glycolysis and the subsequent stages of cellular respiration. Each turn of the cycle processes one molecule of pyruvate, and since glycolysis produces two pyruvate molecules per glucose, the cycle completes twice for every glucose molecule consumed.
3. Oxidative Phosphorylation: Harnessing Electron Power for ATP
Oxidative phosphorylation, the final stage of cellular respiration, takes place in the inner mitochondrial membrane. This aerobic process involves two interconnected components: the electron transport chain (ETC) and chemiosmosis.
Electron Transport Chain (ETC):
High-energy electrons from NADH and FADH2, produced in glycolysis and the citric acid cycle, enter the ETC. As electrons move through a series of protein complexes, energy is gradually released. This energy is used to actively transport protons (H+) across the inner mitochondrial membrane, establishing an electrochemical gradient.
Chemiosmosis:
The electrochemical gradient created by the ETC drives protons back into the mitochondrial matrix through ATP synthase, a molecular turbine. This movement powers the synthesis of ATP from adenosine diphosphate (ADP) and inorganic phosphate (Pi), a process known as chemiosmosis.
Oxidative phosphorylation is highly efficient, generating the majority of ATP in cellular respiration. The final electron acceptor in the ETC is oxygen, forming water as electrons combine with protons and oxygen molecules. This connection to oxygen underscores the aerobic nature of oxidative phosphorylation.
4. Regulation and Control of Cellular Respiration
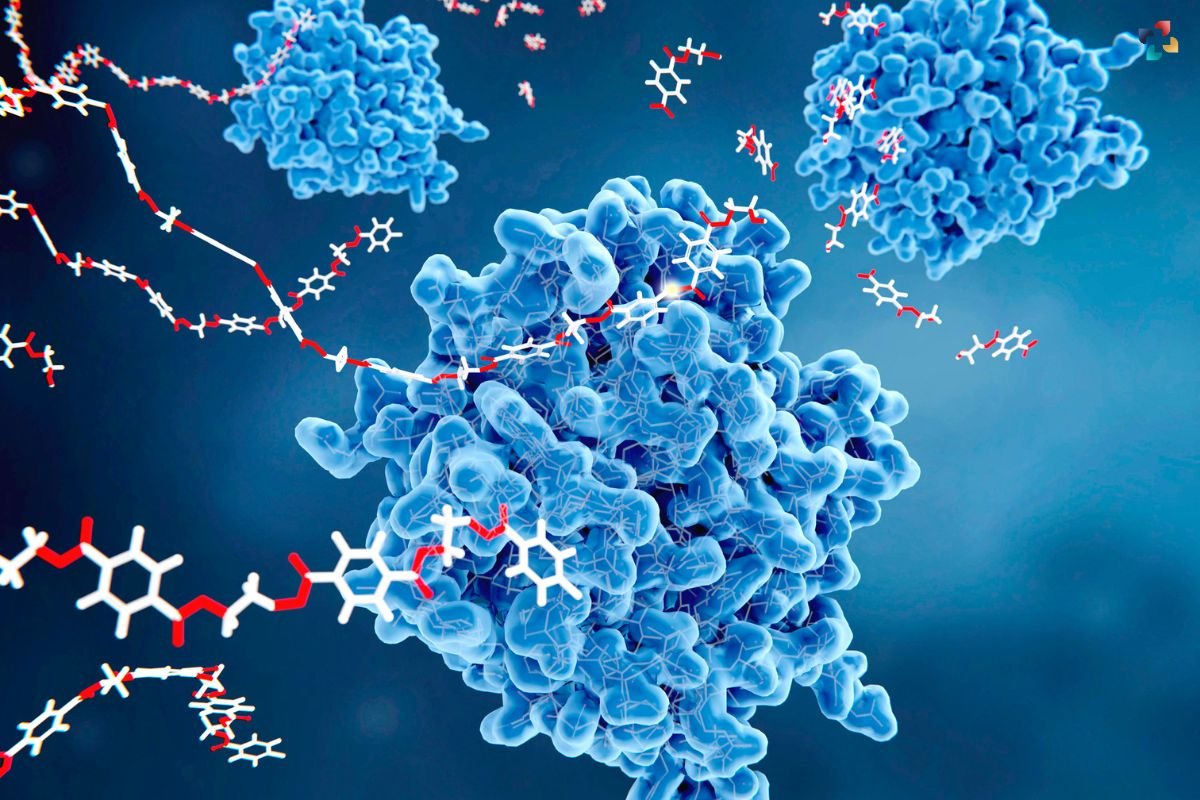
The regulation of cellular respiration involves intricate control mechanisms to adapt to cellular energy needs and environmental conditions. Key regulatory points include allosteric enzymes, feedback inhibition, and the availability of substrates. Adenosine monophosphate (AMP) and adenosine diphosphate (ADP) act as indicators of cellular energy status, influencing the rate of respiration.
Moreover, it responds to the type of nutrients available. Carbohydrates, fats, and proteins can all serve as energy sources, and the metabolic pathways leading to their breakdown converge at different points in the respiration process.
5. Cellular Respiration in Perspective: Beyond ATP Production
While the primary function of cellular respiration is ATP production, its implications extend beyond energy generation. The intermediates produced during the breakdown of glucose are utilized in various biosynthetic pathways, contributing to the synthesis of essential molecules such as amino acids, fatty acids, and nucleotides.
Additionally, cellular respiration intersects with other metabolic pathways, creating a complex network of interconnected processes within the cell. The crosstalk between glycolysis, the citric acid cycle, and biosynthetic pathways enables cells to balance energy production with the demands of growth, maintenance, and reproduction.
Also Read: The Secret Potential of Gut Bacteria: Adapting to Oxygen-Free World
6. Challenges and Adaptations in Cellular Respiration
Cells face challenges that can impact the efficiency of cellular respiration, such as variations in oxygen availability and metabolic demands. In hypoxic conditions, cells may resort to anaerobic respiration, producing ATP through glycolysis without oxygen. While this allows for short-term energy production, it results in the accumulation of lactate or ethanol.
Certain organisms have evolved unique adaptations in cellular respiration to thrive in specific environments. For example, some extremophiles can carry out anaerobic respiration using alternative electron acceptors like sulfate or nitrate, showcasing the versatility of metabolic strategies.
7. Diseases and Disorders Linked to Cellular Respiration
Disruptions in cellular respiration are implicated in various diseases and disorders. Mitochondrial dysfunction, characterized by impaired oxidative phosphorylation, is associated with neurodegenerative diseases, metabolic disorders, and aging. Genetic mutations affecting components of the ETC or mitochondrial DNA can lead to energy deficits and cellular damage.
Moreover, cancer cells often exhibit alterations in cellular respiration to support their rapid growth and proliferation. The Warburg effect, observed in many cancer cells, involves a shift toward glycolysis even in the presence of oxygen, highlighting the metabolic adaptations associated with tumorigenesis.
8. Future Perspectives: Advancements in Cellular Respiration Research
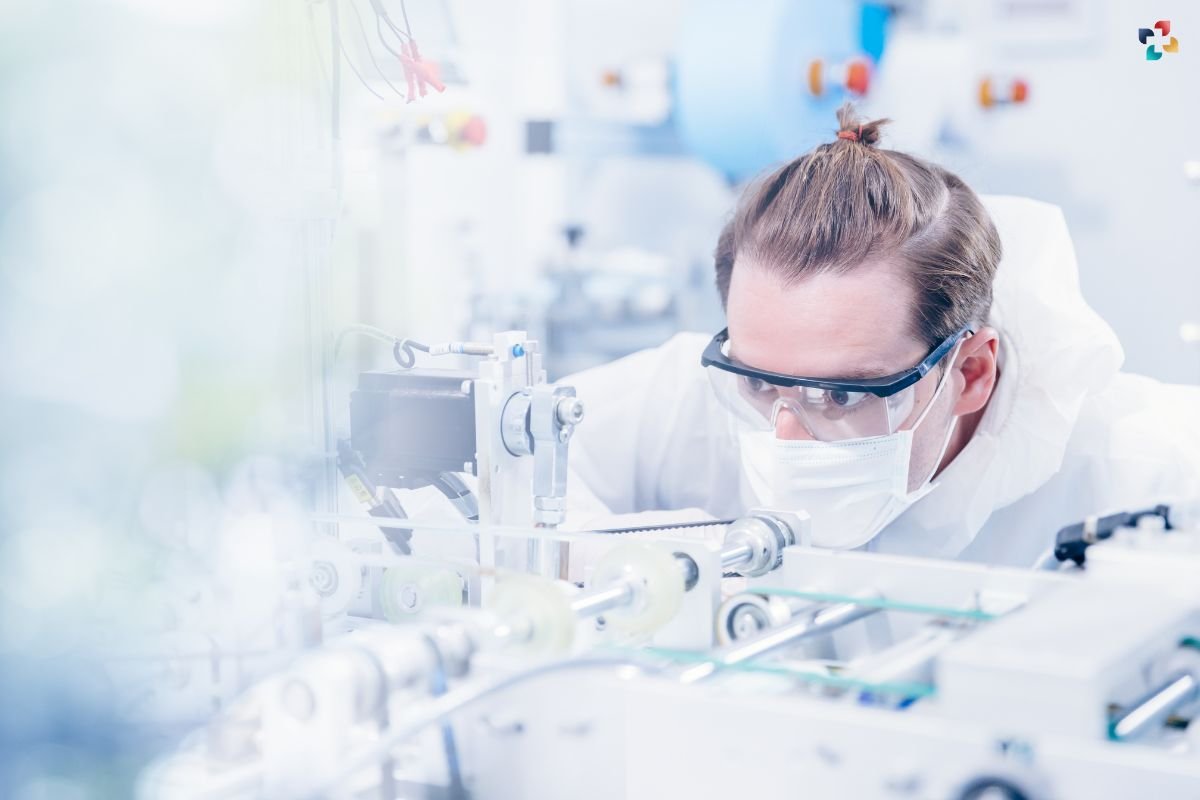
Advancements in technology, such as high-throughput omics approaches and advanced imaging techniques, continue to deepen our understanding of cellular respiration. Researchers explore the roles of newly discovered proteins, regulatory pathways, and mitochondrial dynamics in shaping cellular energy metabolism.
Targeting cellular respiration for therapeutic interventions is an active area of investigation. Mitochondria-targeted drugs, including those aimed at enhancing oxidative phosphorylation or mitigating mitochondrial dysfunction, hold promise for treating diseases associated with metabolic imbalances.
Conclusion: Cellular Respiration as the Energy Hub of Life
Cellular respiration is essentially the central source of energy for all of the various processes that take place inside cells. The process of turning food into ATP, from the earliest routes of glycolysis to the complexities of oxidative phosphorylation, is evidence of the evolutionary success of cellular life.
Knowing about cellular respiration helps to clarify the methods of energy production as well as the connections between various cellular functions. Advances in the study of cellular respiration have great potential for developing novel approaches to disease management, maximizing the generation of bioenergy, and deciphering the profound molecular details of life.